Why do we perform fluid resuscitation?
Shock is defined as a pathophysiological state that occurs when oxygen delivery is insufficient to maintain aerobic respiration in tissue. This is often (but not always) a result of dropping cardiac output. We divide shock into etiological categories, which is important for nuanced treatment, but the physiological effects are very similar in nearly all cases. Today we will be focusing on the types of shock which respond well to fluid administration: Hypovolemic and Distributive. Other types of shock, such as obstructive shock caused by pneumothorax must be rapidly recognized and treated appropriately.

Cardiac output is the volume of the left ventricle (stroke volume) multiplied by the number of times the ventricle pushes blood out each minute (heart rate). Left ventricular volume is around 150mL, but only a fraction (60-70%) of that is ejected with each contraction. Heart rate effects filling volume, volume affects contractility, contractility affects ejection fraction (EF). Failure to maintain a normal CO of 4-8L/min will result in lower blood pressure, impaired cellular metabolism, organ failure, and death.
Blood pressure is the amount of blood being pushed out of the heart (CO) versus how much the vasculature of the body is pushing back (systemic resistance).
Compensation for Shock
Hypovolemic shock is caused by a significant loss of circulating blood volume. This can be caused by bleeding, fluid shifts from burns, sweating, excessive urination caused by disease or medication, or persistent diarrhea. You generally need to lose about 15% of your intravascular volume to start your compensatory mechanism.
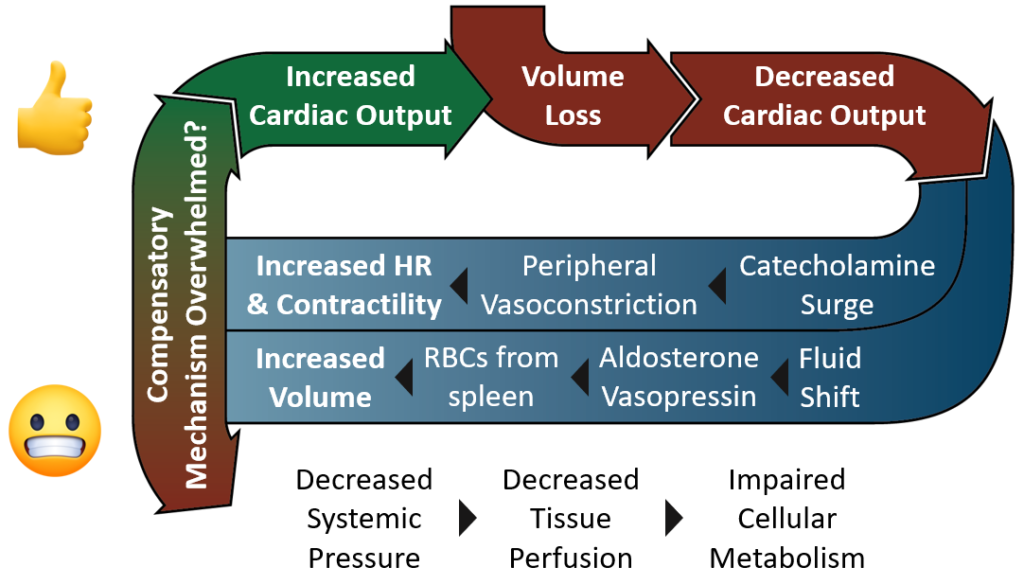
Your body has two means of bringing CO back up: increase heart rate, and increase stroke volume.
Heart Rate
In response to trauma or illness, your adrenal glands release a surge of catecholamines, hormones such as dopamine, epinephrine, and norepinephrine (sympathoadrenal activation). These hormones not only cause the heart to beat faster and harder, but they constrict peripheral blood vessels increasing systemic vascular resistance (SVR).
Volume
Fluid shifts from the interstitial space into the vascular space. The liver and spleen disgorge plasma and young red blood cells. The kidneys release aldosterone which causes retention of sodium (and thus water). The pituitary gland releases Vasopressin, also called antidiuretic hormone or ADH, which causes water to be reabsorbed into the blood from the kidneys. Vasopressin also helps increase SVR by constricting blood vessels.
However, if fluid loss continues the body’s compensatory mechanism will be overwhelmed resulting in CO continuing to drop. Systemic pressures continue to decline which impairs the delivery of oxygen and nutrients to the tissues of the body. Without oxygen and nutrients, cellular metabolism becomes anaerobic resulting in lactic acidosis and electrolyte abnormalities.
Shock Dose
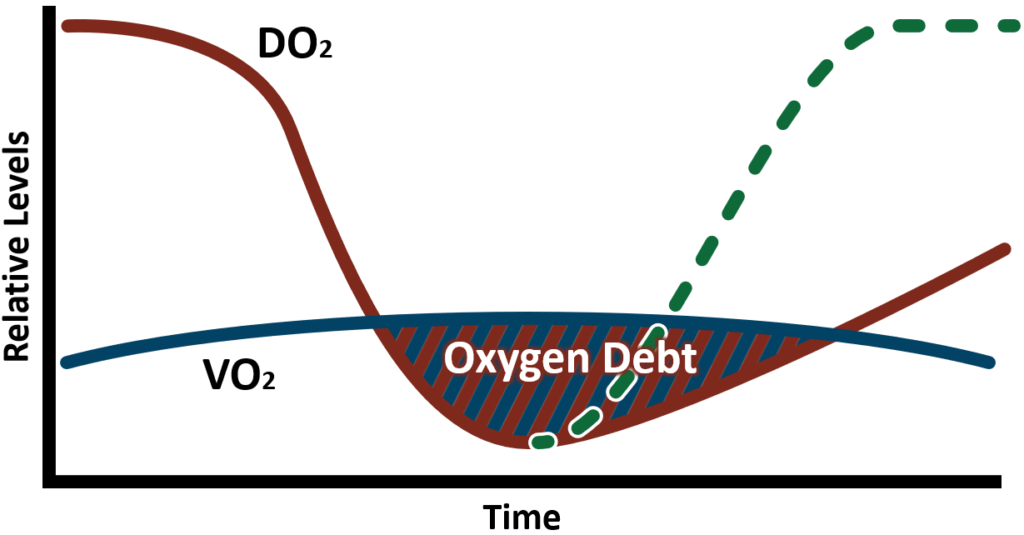
As cardiac output (CO), blood pressure, and by extension delivered oxygen (DO₂) decreases, the rate of tissue oxygen consumption (VO₂) will remain relatively constant as oxygen extraction from red cells increases. However, this only lasts for so long before DO₂ eventually fails to meet VO₂. The point at which those lines cross is when anaerobic metabolism and the accumulation of an oxygen deficit begins.
Oxygen deficit is the difference between the amount of oxygen needed to meet metabolic demand (VO₂) and the amount of oxygen that is being delivered (DO₂). An oxygen deficit occurs when less oxygen is being delivered relative to the required aerobic metabolic demand (shock). Oxygen debt is the accumulation of an oxygen deficit over time. Oxygen debt can be viewed as a quantitative measure of whole-body ischemia. A simple corollary at an individual organ level would be a stroke or acute myocardial infarction where the greater the ischemic burden over time, the greater the final tissue damage and function during and after reperfusion.
Oxygen debt must be repaid quickly. The more debt allowed to accumulate the lower the survival likelihood and the great the number and severity of long-term disability and illness.
Effects of Shock
Assuming that the shocked patient will survive their ordeal, we expect to see long-term consequences or sequelae. These patients should expect life-long low-level organ dysfunction and immunocompromise. This arises from many different factors. Two of them are MODS and SHINE.

Multi-Organ Dysfunction Syndrome (MODS)
The linkage between oxygen debt and traditional organ failure is well recognized and documented. As I mentioned before, the analogy is to a whole-body stroke as ischemia sets in. The organ systems which are most sensitive to accumulated oxygen debt are the brain (stroke), heart (MI), lungs (ARDS), kidney (AKI), liver, and gut (abdominal viscera).
Shock Induced Endotheliopathy
A commonly overlooked complication of shock occurs in the lining of the blood vessels where oxygen, waste, nutrient, and fluid exchanges take place, called the microvasculature. The lining of these micro-vessels is called endothelium. The endothelium is suspected to regulate a variety of processes in the body including the integrity of the blood-organ barrier, clot formation, fluid balance, aspects of immunity, and more. Endotheliopathy is the dysfunction of this critical and widespread regulatory tissue. Shock-Induced Endotheliopathy (SHINE) results in a constellation of counterproductive problems including increased vascular permeability causing fluids to more easily leak out of the vascular space, and the release of anticoagulant hormones preventing the production of clots. Additionally, tiny tree-like structures of the surface of these endothelial cells known as glycocalyx are shed leading to irreversible damage which contributes to long-term endotheliopathy.
How do we perform fluid resuscitation?
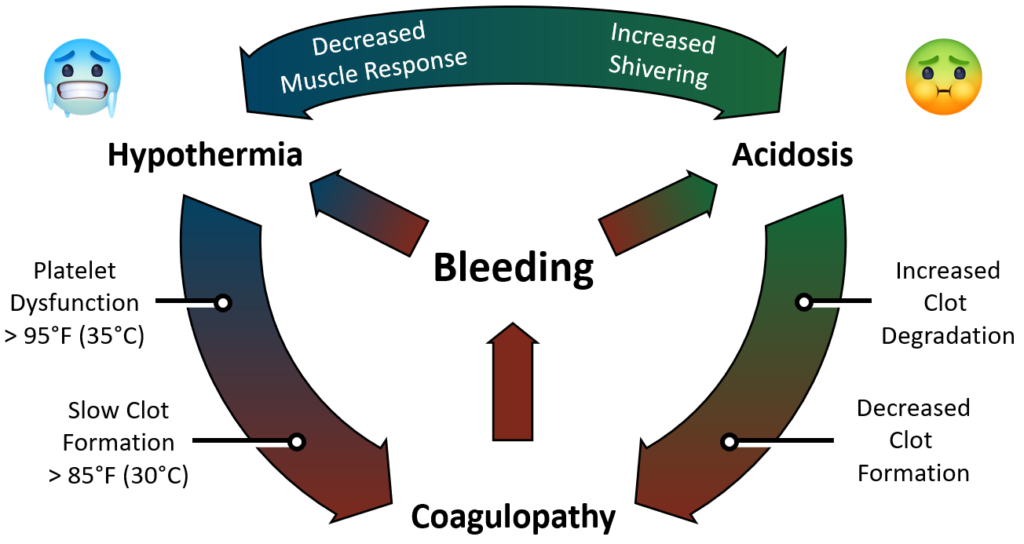
The Lethal Triad is a self-perpetuating cycle of homeostatic disruption which is started by sudden significant volume loss (usually hemorrhagic). Once started, the body quickly goes into a spiral of disfunction. The three parts of the triad are Hypothermia Acidosis both contributing to one another and both leading to increased clotting dysfunction called coagulopathy.
Blood acts as a sort of radiator fluid, transporting thermal energy from heat-producing organs to the rest of the body maintaining body temperature within a very narrow range. If the human body deviates more than a single degree from normal temperature (37°C) we begin to feel very poorly. If the temperature deviates enough, the chemical reactions inside our body slow down and eventually stop. Platelets become less sticky and the production of clots slows down to a standstill.
As a patient loses blood, they lose the ability to supply oxygen to the tissues and cells of the body. The inability to perfuse tissue is called shock. When the cells are unable to get enough oxygen they switch from clean aerobic metabolism over to dirty anaerobic metabolism. The result is that instead of producing lots of energy, a bit of water, and a bit of carbon dioxide, the cell is producing only a little energy and a lot more lactic acid. As the blood becomes more acidic (lower pH) existing clots begin to break down and new clots do not form. Bleeding continues.
As we get cold and begin to shiver, our muscles demand more oxygen to produce movement and heat. This increased metabolic demand without available oxygen which increases lactic acid production. The more acidic the body gets, the less your muscles will activate in response to signals to shiver. More acidosis. More hypothermia. More bleeding.

So how do we interrupt this deadly cycle?
Treatment involves stopping the source of volume loss. STOP THE BLEED! Use all available methods to mechanically control bleeding. Keep every drop of blood you can inside the body. Optimize oxygen delivery to those cells. Prevent heat loss and actively rewarm. Hypothermia and coagulopathies commonly complicate the treatment of hypovolemic shock and are often brought about in part by the provider (iatrogenic).
Replace the lost volume to sustain blood pressure and perfusion. If shock resulted from hemorrhage, whole blood should be used for volume replacement. If tissue perfusion isn’t restored quickly, systemic inflammation and multiple organ failure are likely. Even if the patient survives, they may experience a life of immunocompromise and low-level organ dysfunction.
Goal-Directed Resuscitation

Resuscitation should be performed to a well-defined set of goals. In this case, vital signs. Heart rate, blood pressure, and oxygen saturation are obvious choices. Your local protocols will dictate which vitals are acceptable thresholds for resuscitation. Generally speaking, a reasonable set of vital signs to achieve are: systolic blood pressure (SBP) of 100 mmHg or a mean arterial pressure (MAP) of 65 mmHg; a heart rate of 100 beats per minute; and a saturation of peripheral oxygen (SpO₂) of greater than 93%. Keep in mind the limitations of pulse oximetry in shock states. Measurement of the saturation of tissue oxygen (StO₂) is becoming a more reliable measure of tissue perfusion.
Measurement of lactate levels is a good indicator of the depth of shock and is increasingly available in the field. In a setting where vital signs monitoring is limited, a practitioner can use the level of mentation and strength of radial pulses as an indicator of resuscitation success.
Systemic hypotension with impaired end-organ perfusion necessitates the restoration of intravascular volume to a level sufficient to provide perfusion, but not an attempt to restore normal blood pressure.
What do we use to perform fluid resuscitation?
Crystalloids, and particularly 0.9% saline solution, are by far the most common fluids used for volume replacement both in the prehospital setting and in the emergency department. Other, more balanced crystalloid solutions exist and are seen to varying degrees.
0.9% “Normal” Saline. The pros and cons of 0.9% saline have been expounded upon in recent years. To summarize, there is nothing normal about “normal” saline. The sodium content in a liter of saline is 900mg, or about 30 bags of potato chips. The concentration of sodium in saline is 154 mmol/L which is close to the normal concentration in human blood of 135-145mmol/L. However, to each sodium atom is bound a chlorine atom (also 154 mmol/L) which is significantly higher than the physiological norm of 95-105 mmol/L. This has the potential to create what is called an anion gap acidosis. To compound this, saline is acidic at around 5.5 pH. A normal healthy person can probably receive a liter or two of saline and be just fine. Sick patients, particularly traumatized patients do very poorly when given large amounts of saline.
Lactated Ringer’s Solution. Ringer’s or LR is a saline solution with added electrolytes such as potassium (KCl), calcium (CaCl), and sodium lactate. LR is slightly less acidic than saline, but when administered to a healthy person the sodium lactate is metabolized into sodium bicarbonate. You may recall that bicarbonate is alkaline. This makes LR a more balanced solution. LR is incompatible with some medication, however further studies show that this is less of an issue than once believed.
Plasmalyte-A. Plasmalyte is a balanced crystalloid solution with a pH of 7.40 and an electrolytic composition which exactly matches human plasma. It is significantly more expensive than saline or LR, and doesn’t have any clotting or oxygen-carrying capability.
Blood
Shock resulting from blood loss is best treated with the repletion of blood. In recent years pre-hospital administration of blood products has gone from a laughable concept to one that has been successfully implemented in EMS systems across the nation.
Whole Blood is blood that hasn’t been separated into its constituent parts (RBCs, Plasma, platelets, and clotting products). It is the only truly balanced fluid. Talking about blood types and transfusion reactions are beyond the scope of this talk, but I’d be happy to regale anyone with the specific advancements made by the U.S. Army since 2011.
Blood products have a major logistical hurdle: storage. Most products must be stored at around 39F (4C). To date, there have not been any successful blood analog solutions. However, freeze-dried plasma has been used for decades in other countries and has been used by the Armed Forces for the last ten years with great success. Recently the FDA gave provisional approval for the manufacture and use of FDP for use by the military. Prehospital plasma is associated with a survival benefit when transport times are longer than 20 minutes. We may see shelf-stable reconstituted plasma available for use on our ambulances within the next few years.
Further Reading
Check out the new Damage Control Resuscitation Clinical Practice Guideline available from the Joint Trauma System at jts.amedd.army.mil.